Biomaterials

Proteins interacting with polymer brushes
The control of physico-chemical properties of biointerfaces
Biointerfaces are key to the control of the cell response to biomaterials. In particular, cell adhesion to biomaterials and uptake of nanoparticles is regulated by the biochemical and physico-chemical properties of biointerfaces. Polymer brushes, polymer chains tethered at one end only to an underlying substrate, are particularly interesting coatings to control such biointerfaces. Indeed, these coatings, via the control of their thickness, chain density and the chemistry of their constitutive repeat units, allow tailoring the physico-chemical properties of biointerfaces. As polymer brushes can now be coupled to a wide variety of surfaces, from metals and ceramics to polymers, they allow the tuning of bioactive properties of a wide range of materials, irrespective of their bulk and surface chemistry.
Our work in this area focuses on the functionalization of polymer brushes with cell adhesive peptide sequences, proteins and antibodies engaging cell membrane receptors. We design chemical tools and investigate their efficiency in benign conditions compatible with in vitro cell culture and in vivo work. We study protein adhesion and interaction to polymer brushes, whether in the case of strongly protein and cell-repellent brushes or to optimise binding and immobilisation. Finally we investigate the biofunctional properties of the biomolecules coupled to polymer brushes. This work has implication in the design of coatings for implants, in vitro cell culture systems (in particular for cell nano- and micro-patterning) and for the design of gene delivery vectors.
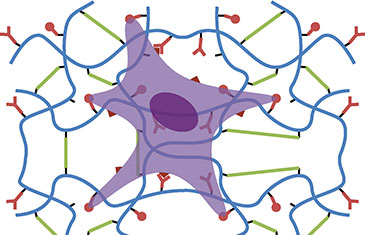
Design of artificial extra-cellular matrix
Peptide-based hydrogels
The extra-cellular matrix plays an important role in the determination of cell phenotypes such as the proliferation of stem cells and their differentiation in defined lineages. Several key features are required to mimic such properties: 1. Provide a mechanical scaffold; 2. Engage specific cell adhesion receptors; 3. Allow matrix remodelling via enzymatic degradation and deposition of new extra-cellular matrix; 4. Sequester cytokines and growth factors involved in cell signalling. Artificial extra-cellular matrix should address these different aspects. To this aim, hydrogels represent a particularly attractive class of materials as they closely mimic and the structural and mechanical properties of extra-cellular matrix in vivo. However, synthetic hydrogels lack the enzymatic degradability, the ability to engage specific receptors on the cell membrane or recruit growth factors and cytokines. Hence, hydrogels based on short peptide sequences conferring such properties are clearly required to confer biofunctionality and a suitable mechanical environment simultaneously.
The design of such peptide-based hydrogels requires a full understanding of the coupling and gelation chemistry to confer the control of the bioactivity of the hydrogels, but also their mechanical properties. Thiol-ene chemistry is emerging as a promising candidate for such applications. It relies on the radical-initiated addition of a thiol to an alkene moiety. This is a particularly well-suited tool for the generation of peptide-based biomaterials as thiols can be conveniently introduced on peptide sequences via cystein residues, whereas alkenes, which do not occur often in the structure of natural biomacromolecules, confer specificity of coupling with a polymer backbone of synthetic or natural origin. In addition, the ability to control such reaction with light (from UV sources to red light, depending on the initiator) makes it well suited for the micropatterning of hydrogels and in situ gelation of cell-loaded scaffolds. Our work in this field focuses on the understanding of the underlying chemistry in physiologically relevant conditions as well as molecular design for applications in tissue engineering.

Patterning tools to structure biomaterials at different length scales
Controlled geometry is an inherent feature of tissues and organs in vivo. The geometrical structure of cells and tissues at multiple length scales is essential to their role and function, but also a hallmark of diseases such as cancer. In vitro, where cells are culture on polystyrene dishes, this control of geometry is typically lost. Reconstructing and controlling some of the geometrical features of biological systems in vitro is an important element of the development of tissue engineering scaffolds and the design of cell based assays. It will allow a better control of cell phenotype, but also higher degree structure and function, which is essential for tissue repair. In addition, it will allow the design of the next generation of cell-based assays, allowing more accurate quantification of cell and organoid behaviour in vitro and determination of the efficacy of drugs and treatments.
Our work has developed the use of patterning tools at different length scales. These patterning techniques have to be fully compatible with the chemistry of the biomaterials on which these platforms are based, as well as the relevant cell culture conditions. At the nano-scale, our work focuses on the use of protein and cell-resistant polymer brushes to control the size and geometry of adhesive patches (generated via colloidal lithography or electro-spinning). At the micro-scale, we use micro-contact printing to generate polymer brush-based protein patterns to which cells will adhere. A the micro- to macro-scale (100 μm to 1 mm), we use combinations of micro-contact printing, hydrogel patterning and microfluidic systems to control the formation and organisation of cell clusters, organoids and micro-tissues.